Agriculture
The History of Evolution: from Darwin to DNA

The Malthus Problem
Almost everyone reading this will have had somewhere between a great grandparent or great great great grandparent who was alive when Darwin went on his legendary trip to The Galapagos Islands in 1831. Many people believe that Darwin came up with the idea for evolution on that trip, but the reality is that the theory predated his birth. What Darwin is famous for is a revolutionary new way of thinking about that idea.
In 1798, Thomas Malthus (of Malthusianism fame), figured out that the productivity of agriculture also meant a rise in the birthrate. On a finite planet, he could see no way that our food supplies would be able to keep up with our increase in population. The more we had to eat the more we had children and the math was not adding up to good news.
Humanity hitting a wall of that sort was a surprise at the time, because people back then saw the world the same way a lot of people still see it today. They believed that nature was growing toward a kind of perfection, like a tree reaching for the light –with humans, like a star, right at the top. But what did it mean if food security –humanity’s greatest success– was also our greatest threat? What did it mean to be successful if success could kill you? Just what light was this tree of nature striving toward?
It was in answering that question that Darwin had his big realization.
Darwin’s Genius
Most people saw evolution the same way most people today understand the idea of so-called pesticide-resistant ‘superweeds.” Most people imagine those weeds as the product of nature actively mutating around our efforts to control them. They imagine the plants intentionally changing in pursuit of survival, which means they imagined that the phrase survival of the fittest meant the ‘survival of the smartest and strongest.’
Darwin saw it for what it really was: animals and plant species sought life, but they weren’t striving to survive –they cannot imagine their future. Their subtle variances simply meant that some simply do survive. Having no foresight, all plants just do what they do, and are what they are. Sometimes their conditions are favourable to their survival and other times they are not, which is why 99.9% of species that have ever existed have gone extinct. With every species, time eventually wins.
A thirsty plant during a drought will not see its genes go forward, nor will a plant that prefers dry soils do well during rainy periods. So rather than nature being a tree, striving upward in search of ever and ever brighter light, Darwin’s big insight was that nature is simply a huge collection of lottery tickets where at least some forms of life are bound to win. And humans are not outside of that fact of nature.
While we’re all enormously alike, parents are always mixing DNA that has never been mixed before. Sometimes mutations –or the mixes themselves– create diseases or weaknesses that weaken or kill us. Other times, we are one of the few genetically lucky lottery winners to survive something like The Spanish Influenza –or, if we’re a weed, survive a farmer’s herbicide. In fact there are no super-weeds, or super-people, there are simply weeds and people that best suit the conditions they happen to be in. In the case of the aforementioned flu, the young and the old were the ones spared while often times it was those in the prime of their lives that did not survive.
Of course, winning this genetic lottery means that the surviving DNA gets to breed more of the subsequent generations. Taking that idea in the opposite direction; Darwin realized that it meant that every living thing was somehow derived from a common ancestor. This was a revolutionary idea at the time.
The churches at the time found these ideas threatening because they created a scientific form of slow-motion creation over which the church had no authority. But for science it was a slowly-evolving eureka moment. To them Darwin’s notion wasn’t dispelling creation, it was seeing it more deeply: fifty percent of the human genome is shared with bananas. That fact does feel like a miracle, and it adds a whole new meaning to the phrase, ‘we are what we eat.’
Of course none of this explained the mechanism by which nature accomplished these variations, nor could we know that the answer might resolve Malthus’s concerns about population.
The Discovery of Genes
Fortunately, in the 1840’s, not long after Darwin’s trip to the Galapagos, a meticulous scientist and monk (which was common at the time), was in the Czech Republic breeding pea plants. Mendel painstakingly crossbred tens of thousands of carefully prepared plants and then just as carefully studied the results. Over time and repetition he realized that there were both dominant and recessive traits that he could predict in subsequent generations.
Mendel was the first person to even imply the idea of genes –the mechanism by which Darwin’s lottery could be held.
By 1869 we had invented technologies that would allow us to look at living things more closely. That’s when a Swiss scientist named Miescher saw something in the nuclei of cells. He even wondered if it could explain Mendel’s heredity mechanism, but at the time no one saw much value in what would come to be known as DNA and RNA.
DNA was pretty simple stuff, made from a nucleotide alphabet of only four letters. But each of our cells contains about two meters of it and we have over ten thousand trillion cells. That’s literally about 20 million kilometers or 12½ million miles of DNA in each of us! If nature’s bothering to create all of that, there’s a reason. But what? It’s only made of four nucleotides. What could you possibly create with a four letter alphabet?
The Colour of Chromosomes
Chromosomes were discovered in 1888, primarily by a German named, Boveri. They got their name because they were really good at absorbing dyes, which makes them easy to see under a microscope (when a cell is dividing). Boveri linked them to the idea of heredity but it was the 1900’s before anyone else really studied them in an effective way.
Thomas Morgan is the reason why so many people associate fruit flies with science experiments. The flies bred so quickly that they were perfect for studying how chromosomes might be affecting heredity. Morgan did for the flies what Mendel did for the peas. And thanks to a mutated fly with the wrong coloured eyes, he was able to track inheritance to the point where many scientists were prepared to work from the assumption that chromosomes and DNA were in fact somehow involved in heredity.
Morgan won a Nobel Prize for his work with the flies, but even 30 years later there were still a lot of people who did not believe genes existed, or that DNA was all that important.
It was about 110 years after Darwin’s voyage on the Beagle, near the end of WWII in the 1940’s, before a brilliant Canadian named Oswald Avery managed to change a bacterium by intentionally introducing a trait from a different bacteria’s DNA. It was that experiment that very cleverly proved to everyone that DNA did in fact explain heredity –and it was so ingenious that there were many who felt Avery deserved two Nobel’s for proving it.
The Shape of Things to Come
With Avery’s discovery made, the race was on to explain DNA’s structure and to understand how it does what it does. If they could figure out the shape of a DNA molecule then science had a better chance of figuring out what it was doing. At the time, it was like trying to figure out how the pieces bolted together to make a bio-machine that made…us.
Many expected the brilliant Linus Pauling to be first the one to figure it out, but maybe knowledge acted as a form of blindness in that case. The people who did find it were fairly unlikely –they had come from a background of working on military weapons. Crick of the famous Watson and Crick didn’t even have a doctorate at the time, although his effort to get one would play a key role in their discovery.
Watson was like a Doogie Howser character –a child genius who had played a role on a popular radio game show. The problem was, he wasn’t very familiar with chemistry. Yet he and Watson’s found themselves trying to figure out how that little four-letter alphabet could be assembled into life. It’s why their discovery was as surprising as it was incredible.
Maurice Wilkins shares the Nobel Prize with Watson and Crick. He is the often-forgotten New Zealander who did a lot of the less glamorous work in developing X-Ray Crystallography that lead to the ability to take images of DNA. That was clearly going to help because, at the time, everyone was following Pauling’s lead –so they were working from the assumption that the DNA molecule’s shape was a triple helix.
The Woman Who Saw Things Clearly
Rosalind Franklin was the woman who figured how to actually take the pictures that Wilkins had theorized, but it was actually a student of hers (named Ray Gosling) who took the now-famous Photo 51. Gosling ended up being moved to work with Wilkins, who many feel shouldn’t have unilaterally showed Franklin’s images to Watson and Crick. But, having seen the image, they could now get their G’s C’s T’s and A’s into a double helix that led Watson and Crick to entirely re-think what they were doing.
Soon after, Franklin wrote a report on an even more detailed photo. That got passed from group leader to group leader at Cambridge until it eventually found its way to Watson and Crick. Using some impressively complex math developed for Crick’s PhD thesis, the two men now used Franklin’s measurements (without her knowledge), and they got the ‘ladder’ of the DNA lined up in such a way that it did produce the proteins that combine to form every living thing. This was an enormous eureka moment, as they say.
(You can actually help science by playing an on-line game called Fold it where you fold those resulting proteins in ways that can help science and humanity. The gamers who do so even get their work into respectable Journals like Nature.)
The reason Franklin went unmentioned for the Nobel was because applying complex math to a photo is easier than creating the complex math to apply to a photo. But had Watson, Crick and Wilkins not beat her to the solution she would have got the answer shortly thereafter, and she was the first person to realize that our DNA forms the subtle variances required to ensure our unique genetic codes.
There was a lot of sexism at the time and that likely played a role Franklin being overlooked but, in the end, even Watson –who had treated her quite badly– admitted so, and regretted that she had died shortly thereafter, preventing him from making proper amends. And of course the Nobel Prize is not given posthumously….
As for the DNA itself, once it was solved it looked easy. The verticals on the DNA ladder are a sugar, and the rungs are the nucleobases we need to make the proteins that fold together to make us. (Drug-based gene therapy is when a drug re-folds an improperly folded protein.) The rungs always have G with C, and T is always with A (unless it’s RNA, then the T is replaced with a U). It’s quite simple chemistry –if you’re a chemist.
In a much more recent development, in the spring of 2018 science was able to confirm a 1990’s theoretical discovery, meaning we also now know there is also i-motif DNA, which is a four strand knot or loop of (C)ytosine to (C)ytosine rungs. (There’s also A, Z, Triplex, Cruciform and G4 DNA shapes, but even scientists don’t know much about what’s going on with those yet, so if you can’t comprehend those you’re in extremely good company.)
After Crick, Watson, Wilkins and Franklin, the next most significant person in our understanding of DNA was the South African, Brenner. In 1960 he figured out that gene DNA is transcribed into messenger RNA in a process called transcription. The translated mRNA transports the genetic information from the cell nucleus into the cytoplasm, where it guides the production of the proteins.
By 1972 a Belgian named Walter Fiers figured out that the parts of our DNA that make the proteins are the genes, and the genes are the sections that organize the proteins to combine into everything a human being is. Shortly thereafter, Herbert Boyer, Stanley Norman Cohen and Paul Berg were the first people to intentionally transfer a gene. Their process got a bacteria to create foreign protein, essentially proving that genetic engineering was possible.
Soon after that, Marc Van Montagu and Jeff Schell found a little circular piece of DNA outside the chromosome of Agrobacterium tumefaciens. In nature it’s a bacteria that put tumors on trees, but they suspected it could also facilitate gene transfer between species in nature. By the early 80’s they had worked the Americans and the French to create the first genetically engineered plant –a variety of tobacco.
In 1974 Rudolph Jaenisch had engineered a mammal, creating the first mouse. That in turn incited a huge shift in medical research because that discovery made it possible to do experiments on exactly the same mouse over and over, which is obviously very helpful in scientific research.
Then, almost miraculously, in 1977, Carl Woese (and George E. Fox) made possibly the least-known yet most important discovery since Darwin himself, when they disproved Darwin’s notion of nature as a ‘tree of life.’ This later set Woese on a path that demonstrated the significance of Horizontal Gene Transfer. That discovery effectively saw Darwin’s ‘tree’ suddenly evolve into a bush –which demonstrated that, just as modern GMOs do, nature did and does move genes from one species to another, with the Sweet potato being a popular example. (Later, our human genome was found to be 8% virus.)
Enter Craig Venter in 2000. He and his team are the first to map the entire human genome. That same technology is now being used to map the genomes of countless plants and animals. It is through these processes that some diseases are discovered that relate to mistakes in copying the DNA code, and that lead to things like cancers.
By 2012, Jennifer Doudna and Emmanuelle Charpentier, only the second and third woman in the bunch, make maybe the most practical discovery in genetics when they figure out how to use a technology called CRISPR to get nature itself to edit or patch genetic code. This process is so natural that if we use it to create a new food it isn’t even considered genetically modified because it comes about the very same way that nature does it.
That takes us to where science is today. But this begs the question, how does DNA actually work?
Cell Splits, DNA Snips and Cancer
When our cells split our 2 meters of DNA comes unzipped down the middle of the ‘ladder.’ But because it’s a code where Cs always link to G’s and T’s always link to A’s, it only takes about a second and nature has made a new piece of matching DNA and you have a whole new ‘ladder.’
We do this unzipping and recreating a lot with our colon cells because they only survive a few days; skin cells maybe a month; and like pretty much all cells, the liver cells get replaced constantly. But each individual one only replicates about once every 11-17 months. This explains why we’re often tired when we’re recovering from surgery. On top of any damage we have to repair, we have about 50-100 trillion cells and about 300 million die every minute, so it’s easy to see that our bodies are very busy.
For the most part these processes go extremely well, but it is possible to have a split go slightly wrong –that’s when a wrong letter gets in the wrong place. Biochemists call that a snip. Snips are how we get mutations that can sometimes give us cancer, and that’s why older people get more cancer. They’ve simply had more cell divisions –or more time for more splits and snips. This also explains why cancers will grow much faster in some parts of the body than in others –it depends on the rate of cell replacement.
Despite the fact that they sometimes can lead to cancer, snips are also what makes each of us just unique enough that some of us survive The Spanish Influenza pandemic while others do not. If you saw the film GATTACA, (so-named for the four nucleotides in DNA), a snip was Ethan Hawke’s advantage in the film.
Too much snipping and we die. Too little and we never evolve. Our existence literally balances between those two opposing concepts, hence our interest in genetic engineering –it’s like tipping the balance in our favour. And now we also tip it in nature’s favour too, which is why we don’t need baby cows for rennet, horseshoe crabs for the antibodies in their blood, or pigs for insulin. And, as an example, if we can get more ears of corn on a single plant, then we can leave more wild spaces for nature.
Conscious Modification
Once we understood that the genes were made of chunks of DNA that simply coded for proteins, we realized that the Natives who turned teosinte grass into modern corn –about 10,000 years ago– were actually doing a valuable yet blindfolded form of genetic engineering.
On a modern level, despite the fact that Darwin had pointed out that we are all descended from one species (about 3.8 billion years ago), scientists were still surprised when they started noticing that the genes that made a mouse eye for a mouse would amazingly make a fly’s eye on a fly. Before they knew it the scientists realized they –and we– share about 60% of our code with flies! We even have the genes for a tail, that gene just isn’t switched on. It’s both unifying and humbling in a way. All life shares the same interchangeable LEGO, we just build different things with it.
Today, with the help of supercomputers, we can map out the genome of things very quickly. We can also imagine what would be created if you mixed things that haven’t mixed yet because we know what the codes actually do in the plants we improve. This means the beneficial changes created by genetic engineering could have happened in nature, but our advantage is that we do it intentionally, when otherwise a growing population could easily starve while waiting for nature to stumble onto the answers that will feed a future world.
Today’s accurate computer models also allow scientists to avoid wasting time on crops that they can figure out won’t survive, or that may be allergenic, etc. That gives them more time to develop the plants that are fit to be food. If any of these changes seems unnatural, remember, Darwin didn’t actually use the term survival of the fittest to describe evolutionary success –he simply described it as, descent through modification. Genetic engineering is merely conscious, intentional modification.
Working With Nature
When a scientist makes a crop that has an insecticide ‘inside it,’ the insecticide is BT, or bacillus thuringiensis. Much like a bacteria created a sweet potato by inserting its genes into a potato, BT is a bacteria commonly found in soil that is deadly to certain bugs. It’s the very same BT that organic farmers spray on their crops because their rules mean they are barred from using the GMO BT strains that have the DNA coding to create the BT within the plant itself.
The BT in a GMO is still normal BT, but it’s a part of nature that makes very specific bug’s guts –which are alkaline, not acidic like ours– explode. That’s not dangerous for mammals for much the same reason that your mother doesn’t have to be afraid of Tiger Lilies but she should keep them away from her cat. As with dogs and chocolate, what can kill one species can be irrelevant to another. But both the BT and Tiger Lillies are natural, and BT is a great example of how science can use genetic engineering to protect beneficial insects.
Can humans make mistakes? Yes. They do so quite regularly. But on important things we do a lot of double checking, and our food has never undergone more testing, whereas nature creates random things like poisonous mushrooms etc. Fortunately, genetic engineering has been precise enough for long enough that it is now proving it can generate substantial gains for humans and our environment.
Far from being afraid of the manipulation of DNA, we should see nature as Darwin’s lottery, where nature produces mostly losing tickets. In contrast, genetic engineering permits the wildness of nature to exist while also allowing us to recognize and define the traits that farmers will need when it comes to growing the crops that will sustainably feed a growing world.
Which brings us back to Malthus and his math problem.
Malthus Meets the Green Revolution
What Malthus could or did not include in his calculations were human things like genetically precise plant breeding, mechanization, The Green Revolution (created by plant hybrids and nitrogen fertilizer), as well as advances in soil science, genetic engineering, and satellite-aided precision agriculture. He also didn’t know that education would lower birthrates, which means the population will actually start dropping to a sustainable level starting somewhere between 2050 and 2100.
As recently as 1968 people like Paul Ehrlich were writing best-selling books that made Malthusian predictions that hundreds of millions of people would be starving every year by the 1980’s. That obviously didn’t happen, thanks in large part to genetic science. In fact, there are fewer starving people today than ever before, and most of those are due to war, not any failings of agriculture.
A Rationally Optimistic Future
Humans cannot move forward using ignorance and fear. Our future depends on us proceeding forward with the inventiveness implied by Rational Optimism. We must be realistic, and yet at the same time we must take what we learn about nature and use it to help both ourselves and nature.
We cannot do our best for the environment, for our nutrition, or for feeding the world if we don’t use all of the tools that science has discovered on its march through time. That can be as simple as a Native American putting a fish for nitrogen on a corn seed 5,000 years ago, or a geneticist helping a plant develop drought tolerance in a lab.
In agriculture, and in life in general, humans are simply using what we know in the most productive ways we can find. Our knowledge of DNA, coupled with the love of nature that lead to the existence of the sciences, will be absolutely key to us succeeding in sustainably feeding a growing planet.
Note: If you would like a short shareable video version of this article it can be found here.
Agriculture
Dairy Farmers Need To Wake Up Before The System Crumbles
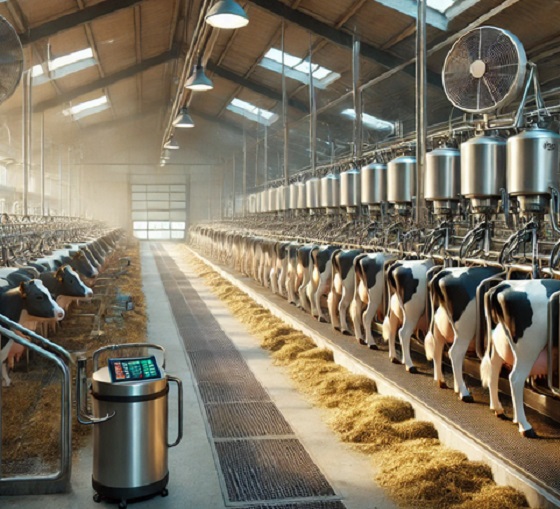
From the Frontier Centre for Public Policy
Without reform, Canada risks losing nearly half of its dairy farms by 2030, according to experts
Few topics in Canadian agriculture generate as much debate as supply management in the dairy sector. The issue gained renewed attention when former U.S. President Donald Trump criticized Canada’s protectionist stance during NAFTA renegotiations, underscoring the need to reassess the system’s long-term viability.
While proponents argue that supply management ensures financial stability for farmers and shields them from global market volatility, critics contend that it inflates consumer prices, limits competition, and stifles innovation. A policy assessment titled Supply Management 2.0: A Policy Assessment and a Possible Roadmap for the Canadian Dairy Sector, conducted by researchers at Dalhousie University and the University of Guelph, sheds light on the system’s inefficiencies and presents a compelling case for reform.
Designed in the 1970s to regulate production and stabilize dairy prices, Canada’s supply management system operates through strict production quotas and high import tariffs. However, as successive trade agreements such as the USMCA, CETA, and CPTPP erode these protections, the system appears increasingly fragile. The federal government’s $3-billion compensation package to dairy farmers for hypothetical trade losses is a clear indication that the current structure is unsustainable.
Instead of fostering resilience, supply management has created an industry that is increasingly dependent on government payouts rather than market-driven efficiencies. If current trends persist, Canada could lose nearly half of its dairy farms by 2030 — regardless of who is in the White House.
Consumer sentiment is also shifting. Younger generations are questioning the sustainability and transparency of the dairy industry, particularly in light of scandals such as ButterGate, where palm oil supplements were used in cow feed to alter butterfat content, making butter harder at room temperature. Additionally, undisclosed milk dumping of anywhere between 600 million to 1 billion litres annually has further eroded public trust. These factors indicate that the industry is failing to align with evolving consumer expectations.
One of the most alarming findings in the policy assessment is the extent of overcapitalization in the dairy sector. Government compensation payments, coupled with rigid production quotas, have encouraged inefficiency rather than fostering innovation. Unlike their counterparts in Australia and the European Union — where deregulation has driven productivity gains — Canadian dairy farmers remain insulated from competitive pressures that could otherwise drive modernization.
The policy assessment also highlights a growing geographic imbalance in dairy production. Over 74% of Canada’s dairy farms are concentrated in Quebec and Ontario, despite only 61% of the national population residing in these provinces. This concentration exacerbates supply chain inefficiencies and increases price disparities. As a result, consumers in Atlantic Canada, the North, and Indigenous communities face disproportionately high dairy costs, raising serious food security concerns. Addressing these imbalances requires policies that promote regional diversification in dairy production.
A key element of modernization must involve a gradual reform of production quotas and tariffs. The existing quota system restricts farmers’ ability to respond dynamically to market signals. While quota allocation is managed provincially, harmonizing the system at the federal level would create a more cohesive market. Moving toward a flexible quota model, with expansion mechanisms based on demand, would increase competitiveness and efficiency.
Tariff policies also warrant reassessment. While tariffs provide necessary protection for domestic producers, they currently contribute to artificially inflated consumer prices. A phased reduction in tariffs, complemented by direct incentives for farmers investing in productivity-enhancing innovations and sustainability initiatives, could strike a balance between maintaining food sovereignty and fostering competitiveness.
Despite calls for reform, inertia persists due to entrenched interests within the sector. However, resistance is not a viable long-term strategy. Industrial milk prices in Canada are now the highest in the Western world, making the sector increasingly uncompetitive on a global scale. While supply management also governs poultry and eggs, these industries have adapted more effectively, remaining competitive through efficiency improvements and innovation. In contrast, the dairy sector continues to grapple with structural inefficiencies and a lack of modernization.
That said, abolishing supply management outright is neither desirable nor practical. A sudden removal of protections would expose Canadian dairy farmers to aggressive foreign competition, risking rural economic stability and jeopardizing domestic food security. Instead, a balanced approach is needed — one that preserves the core benefits of supply management while integrating market-driven reforms to ensure the industry remains competitive, innovative and sustainable.
Canada’s supply management system, once a pillar of stability, has become an impediment to progress. As global trade dynamics shift and consumer expectations evolve, policymakers have an opportunity to modernize the system in a way that balances fair pricing with market efficiency. The recommendations from Supply Management 2.0 suggest that regional diversification of dairy production, value-chain-based pricing models that align production with actual market demand, and a stronger emphasis on research and development could help modernize the industry. Performance-based government compensation, rather than blanket payouts that preserve inefficiencies, would also improve long-term sustainability.
The question is no longer whether reform is necessary, but whether the dairy industry and policymakers are prepared to embrace it. A smarter, more flexible supply management framework will be crucial in ensuring that Canadian dairy remains resilient, competitive, and sustainable for future generations.
Dr. Sylvain Charlebois is senior director of the agri-food analytics lab and a professor in food distribution and policy at Dalhousie University.
Agriculture
How USAID Assisted the Corporate Takeover of Ukrainian Agriculture
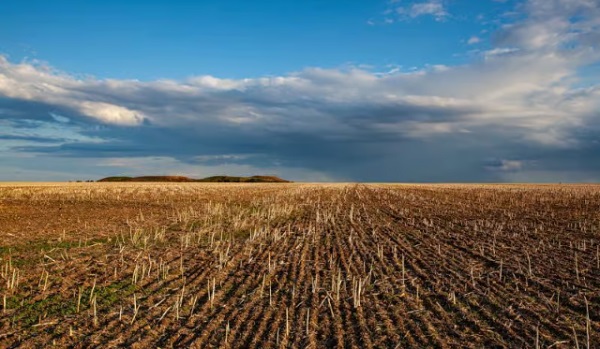
From the Brownstone Institute
By
A recent essay titled “The Real Purpose of Net Zero” by Jefferey Jaxon posited that Europe’s current war against farmers in the name of preventing climate change is ultimately designed to inflict famine. Jaxon is not speculating on globalist motives; he is warning humanity of a rapidly unfolding reality that is observable in the perverse lies against cows, denigration of European farmers as enemies of the Earth, and calls by the WHO, WEF, and UN for a plant-based diet dependent entirely on GMOs, synthetic fertilizers, and agrichemicals.
Revelations about the evil doings of the Orwellian-monikered “United States Agency of International Development” (USAID) reveal a roadmap to totalitarian control unwittingly funded by America’s taxpaying proles. USAID’s clandestine machinations have long focused on controlling local and global food supplies as “soft colonization” by multinational chemical, agricultural, and financial corporations. European farmers revolting against climate, wildlife, and animal rights policies are harbingers of this tightening globalist noose.
The roots of the current globalist plan to “save humanity from climate change” link directly to the infamous Kissinger Report, which called to control world food supplies and agriculture as part of a globalist collaboration between nation-states and NGOs to advance US national security interests and “save the world” from human overpopulation using “fertility reduction technologies.” Kissinger’s 1974 Report was created by USAID, the CIA, and various federal agencies, including the USDA.
Fast forward to 2003, the Iraq War justified using fear-mongering propaganda about weapons of mass destruction and neo-conservative malarky about rescuing the Iraqi people. The US-led occupation of Iraq became a rapacious profiteering smorgasbord for colonizing corporations husbanded by USAID. Iraq is heir to the birthplace of human civilization, made possible by early Mesopotamian agriculture: many of the grains, fruits, and vegetables that now feed the world were developed there. Iraq’s farmers saved back 97% of their seed stocks from their own harvests before the US invasion. Under Paul Bremer, Rule 81 (never fully implemented) sought to institute GMO cropping and patented seed varieties, as Cargill, Monsanto, and other corporations descended upon the war-ravaged nation using American tax dollars and USAID.
That playbook was more quietly implemented during the Ukraine War, once again orchestrated by USAID. Before the Russian invasion on February 24, 2022, Ukraine was the breadbasket of Europe, prohibiting GMO technologies and restricting land ownership to Ukrainians. Within months of US intervention, USAID assisted in the dismantling of these protections in the name of “land reforms,” free markets, financial support, improved agricultural efficiency, and rescuing the Ukrainian people. In just two years, over half of Ukraine’s farmland became the property of foreign investors. GMO seeds and drone technology were “donated” by Bayer Corporation, and companies such as GMO seed-seller Syngenta and German chemical manufacturer BASF became the dominant agricultural “stakeholders” in war-torn Ukraine. Russia may withdraw, but Ukraine’s foreign debts, soil degradation, and soft colonization will remain.
The UN, WTO, WHO, and WEF all conspire to peddle a false narrative that cows and peasant farmers are destroying the planet, and that chemical-dependent GMO monocropping, synthetic fertilizers, and patented fake meats and bug burgers must be implemented post haste (by force if necessary) to rescue humanity. The argument that pesticides and synthetic fertilizers (manufactured from natural gas, aka methane) are salvific is patently false. They are, however, highly profitable for chemical companies like Bayer, Dow, and BASF.
Jefferey Jaxon is exactly correct. The Netherlands committed to robust agricultural development following a Nazi embargo that deliberately inflicted mass famine following their collaboration with Allied Forces in Operation Market Garden. France boasts the highest cow population in all of Europe. Ireland’s culture is tightly linked to farming as part of its trauma during the (British-assisted) Irish Potato Famine. The corporate/NGO cabal now uprooting and targeting farmers in these nations and across the EU in the name of staving off climate change and preserving wildlife is a direct outcropping of Kissinger’s grand dystopian scheme launched through USAID in 1974.
Americans watch European farmer protests from afar, largely oblivious that most all of US agriculture was absorbed by the Big Ag Borg generations ago. Currency control linked to a (political, environmental, and economic) social credit scorecard promises the fruition of Kissinger’s demonic plan: “Control the food, control the people.”
Modern humans suffer a double hubris that blinds them to the contemplation of the truth of Jaxon’s hypothesis: a cultish trust in technology, coupled with an irrational faith in their self-perceived moral superiority to past civilizations (Wendell Berry calls this “historical pride”). Yet, as long as mankind has had the capacity to harm another for personal gain, humans have devised ways to control food for power or profit. Siege warfare generally depended on starving defenders of castle walls into submission.
Even if globalist food control proposals are well-intentioned, a monolithic, monocultured, industrial-dependent worldwide food system is a lurking humanitarian disaster. Berry observed:
In a highly centralized and industrialized food-supply system there can be no small disaster. Whether it be a production “error” or a corn blight, the disaster is not foreseen until it exists; it is not recognized until it is widespread.
The current push to dominate global food production using industrial systems is the cornerstone of complete globalist dominion over all of humanity. The “Mark of the Beast” without which no American will buy or sell goods – including guns, bullets, or factory-grown hamburgers and cricket patties – is mere steps away. Mr. Jaxon is correct that these leaders “know these basic historical and current facts,” and that “[f]armers are becoming endangered because of government [climate] policy … and it’s being allowed to happen.” USAID has been actively seeding and watering this dystopia for decades.
Klaus Schwab and Bill Gates are as fully cognizant of this fundamental truth as Henry Kissinger was in 1974. USAID has aided all three. Having lost almost all of their small farms over the last century, Americans are well ahead of Europeans in their near-complete dependence on industrial food.
That’s the plan.
-
National1 day ago
Trudeau fills Canadian courts with Liberal-appointed judges before resigning as prime minister
-
Censorship Industrial Complex2 days ago
Misinformed: Hyped heat deaths and ignored cold deaths
-
International1 day ago
Commerce Secretary on Oval Office debacle: Zelensky flies to Washington to sign deal then scuttles it
-
Business1 day ago
Trump’s trade war and what it means for Canada
-
Alberta1 day ago
Securing the Alberta-U.S. border
-
Business2 days ago
Trump could announce tariff compromise Wednesday
-
Business1 day ago
Next federal government has to unravel mess created by 10 years of Trudeau policies
-
Crime1 day ago
Reporter Exposes The Left’s $20 Billion Climate Slush Fund